Electronic transformers, inductors and magnetic materials are ubiquitous in many applications, from power adapters, laptops and mobile devices to car electronics, LED lighting, medical electronics and industrial controls. However, the enabling technology remains unsung.
Many designers regard magnetic and electromagnetic components as ‘low tech’, but much technology and know-how is applied to designing and constructing these passive components.
Technological developments – energy efficiency, weight minimisation, surface mounting and miniaturisation – have been driving growth in the sector. Future growth in the markets for electronic coils, transformers and other inductors will be driven by new, high tech electronic components.
Developments in materials, winding techniques and equipment, and design solutions are creating new optimised products across a range of applications.
Design
Practical electromagnetic component design requires knowledge of electrical principles and materials, as well as economics. While small devices such as low-voltage transformers under 10kVA can be designed using handbook data and pencil-and-paper calculations, specialist devices and larger or mass‑produced units require extensive computer-aided modelling.
Even the most sophisticated design and analysis has at its heart the fundamentals of magnetic and electrical circuits – Maxwell’s equations, Ampere’s law, Faraday’s law, Gauss’s law and Lenz’s law. Best-in-class design software takes these fundamentals and applies them to today’s electromagnetic field simulation and modelling with sophisticated computation and visualisation techniques.
For example, Etal harnesses the power of simulation software based on the finite element method to simulate low-frequency electromagnetic fields in a wide range of industrial components. Examples run from 2D magnetic transient, AC electromagnetic, magnetostatic, electrostatic and DC conduction to electric transient solvers. It will solve for field parameters including capacitance, inductance, resistance and impedance.
Once we have the results, we can build a full 3D CAD drawing, and customers can then move forward with the mechanical and electrical design of their system without the need for physical samples of the finished component.
This enables component design teams to create accurate models of magnetic components within the CAD package. They can try out the impact of materials, wires and air gaps, tuning the design to get as close as possible to the parameters required by the customer. Using simulation to tune the design allows optimum performance to be achieved without the need to create multiple prototypes. Designs can integrate new magnetic materials, wires, winding and manufacturing techniques at the earliest possible stage.
Innovative magnetic materials
For the electromagnets used in inductors, transformers, DC-DC converters and the like, designers look for core material that delivers high permeability and maximum flux density. Iron and alloys like SiFe are the traditional starting point.
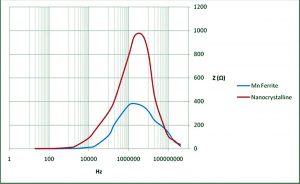
Figure 2: Nanocrystalline common-mode chokes provide damping of noise frequency over a wider frequency range – more than double the range of ferrite aloternatives
Ferrites – ceramic, homogeneous materials – are composed of various oxides. Those with iron oxide as their main constituent exhibit excellent EMI protection against common mode and differential conducted noise, since their insertion loss is proportional to frequency – showing no attenuation to signals, but high impedance to high frequency noise.
For power conversion applications, working temperature, flux density and frequency are the key parameters to select the proper material: from standard 60°C to 100°C in handheld converters to -20°C to 100°C or -60°C to 140°C for automotive or industrial applications.
Converters operate at a wide switching frequency range, depending on voltage, power and cost constraints. Specific materials enable components to operate from just a few kHz through to hundreds of kHz and even MHz, providing efficient, compact converters.
Powder cores are distributed air gap cores that are primarily used in power inductor applications, specifically in switched-mode power supply output filters, also known as DC inductors. Different core materials have particular advantages for certain applications. For lowest loss, core loss is the key factor, while designs requiring minimum core size, such as a DC bias dominated design, should use materials with highest flux capacity.
Saturation is another property to consider, with available materials providing trade-offs between low losses and reasonably high saturation (0.8T) at a low cost, up to higher priced, high saturation material (1.6 T). High saturation is advantageous where inductance under load is critical.
An advance on powder technologies, new amorphous and nanocrystalline magnetic cores allow smaller, lighter and more energy-efficient designs in many high frequency applications for inverters, adjustable speed drives and power supplies.
Amorphous metals are produced by using special technology where molten metal is cast into thin solid ribbons. Since the material has no crystalline magnetic anisotropy, amorphous magnetic metal has high permeability.
When compared with conventional crystalline magnetic materials, amorphous magnetic cores have superior magnetic characteristics, such as lower core loss. They offer superior design when used as the core material.
Nanocrystalline alloys offer a unique combination of high permeability with large flux density and low losses at high frequencies. Operational temperatures of up to 180°C are possible.
Materials like this enable construction of chokes and transformers in much smaller dimensions than is possible using ferrite based assemblies. Common-mode chokes, in particular, benefit from the high permeability, because the amount of copper wire can be reduced.
Wire is important
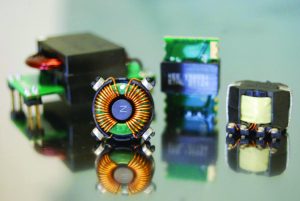
Figure 4: Advanced design and innovative materials bring new possibilities for custom magnetic components
The introduction of triple insulated wire allows windings to be laid on top of each other, giving mains isolation in a smaller form factor. This technique meets all the leading international safety codes and enables transformers to be manufactured to meet safety isolation standards without the need for margins and tape barriers.
With conductor diameters ranging from 0.2mm to 1mm the increased winding space permits smaller transformers to be designed and reduces manufacturing time and cost. Advances in magnetic materials, wire, winding techniques and equipment can be fed back rapidly through accurate simulation tool and 3D CAD solutions.